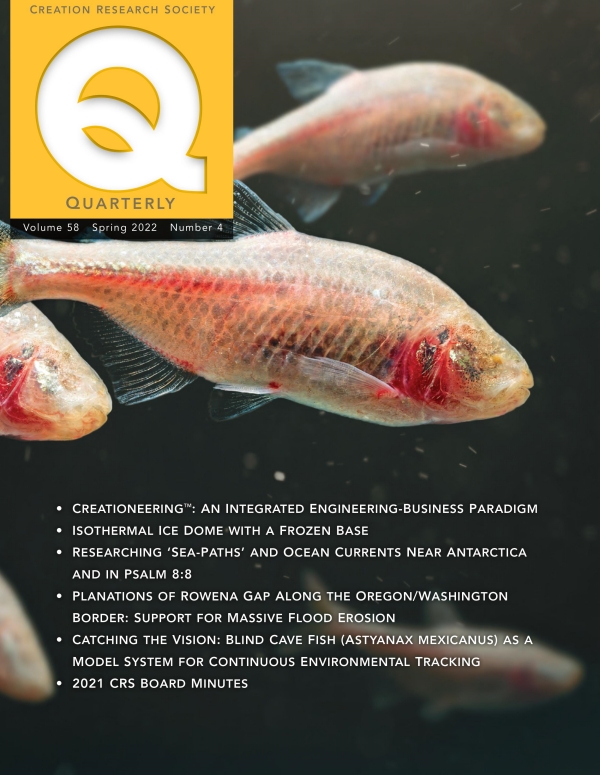
Abstract
A wide variety of fish, amphibians, and arthropods have colonized cave environments deploying complex suites of morphological, physiological, and behavioral traits specifically adaptive to a perpetually dark and resource-scarce environment. Among the many examples of this global phenomenon is the Mexican blind cavefish (Astyanax mexicanus) which has become a leading research model for this rapid and repeatable adaptation which is nearly entirely due to developmental phenotypic plasticity. Not only do blind Astyanax cavefish (compared to surface morphotypes) exhibit a lack of eye development, but they also display complex enhancements for chemo- and mechano-sensors, altered circadian cycles, modified neural and endocrine circuits, metabolic modifications in both rates and metabolism, epigenetic modifications, and behavioral changes that rely upon all of these complex physical and physiological modifications. These rapid, repeatable, and complex organism-wide system adaptations make little sense in the context of Darwinian evolution involving mutation and natural selection. However, the data fit perfectly with a model of engineered, organism-driven systems of adaptation built into creatures by the Creator that enable them to continuously track and appropriately adjust to specific environmental changes. Given that Astyanax have been well-researched and are relatively easily studied, reared, and bred in a laboratory setting, we propose using these fish in creationist research for the study of environmental tracking conferred primarily through phenotypic plasticity.
*Jeffrey P. Tomkins, Scott Arledge, and Randy J. Guliuzza, Institute for Creation Research, Dallas, TX (www.icr.org).
Introduction
It is not uncommon for certain types of animals to deploy unique suites of variable morphological, physiological, and behavioral adaptative traits that allow them to live solely in underground habitats such as caves (referred to as troglobites). These cave-exclusive traits are distinct from those in trogloxenes, creatures that live both above-ground and underground or in caves (e.g., bats), or animals that visit caves. Troglobitic creature variants with cave-specific self-adjustments living in perpetual darkness can be found among a diversity of amphibians, fish, various insects, crustaceans, millipedes, and spiders.
There are about 230 known species of dark-adapted cave fish (CF) across the world and over a nine-year period (2011 to 2020), an average of eight new species per year were documented (Maldonado et al., 2020). All of these CF independently originated from surface dwelling morphotypes, indicating that the trait is a consistently repeatable adaptation (Borowsky, 2018). The most heavily studied troglobitic fish is the blind CF Astyanax mexicanus which has colonized about 30 different caves in northeast Mexico and has become a model organism for perpetually dark ecosystem adaptation (Gross, 2012).
Astyanax has proven especially useful in studying cave adaptation because the cave-dwelling morphotype lives in close proximity to surface-dwelling morphotypes found in lacustrine or fluvial environments. Genetic studies have yielded evidence of multiple cave invasions (five by the latest count) by surface-dwelling types along with persistent gene-flow among cave-dwelling populations (McGaugh et al., 2020a; Maldonado et al., 2020). However, there is a limited amount of overall genetic variability between the surface and cave variants and their genomes are nearly identical. The two different groups (sighted vs. blind) are referred to as morphotypes and not separate species because of their nearly identical genomes. In addition, no inactivating mutations have been found in the genes associated with eye loss (Gore et al., 2018). Because of a paucity of correlating genetic variants as being solely causal to the blind CF morphotype, a large amount of research has investigated and fortuitously revealed a diversity of internal systems leading to the overall CF phenotype – bolstering an engineering-based model to explain the operation of adaptable systems in creatures.
The characteristics that describe how organisms adapt to changing exposures are evidence that could be used to support either an engineering-based theory or a Darwinian theory of adaptation. A good theory of adaptation should try to establish objective means of testing its correctness by making unambiguous predictions of how adaptation should be characterized. A new engineering-based model of adaptation called continuous environmental tracking (CET) postulates adaptation will be best explained by the same engineering principles as a human-engineered tracking system (Guliuzza and Gaskill, 2018). This model predicts that adaptive changes would be characterized as highly regulated, rapid, repeatable, sometimes reversible, and produce solutions that target and may even anticipate specific environmental challenges. Darwinian theory also makes predictions. Stephen Jay Gould detailed why within evolutionary theory both genetic variation and adaptation “must” be characterized as “undirected,” “copious,” and “small in extent,” i.e., very gradual. Gould adds, “Substantial change might occur as a very rare event, but most alteration must be insensible, even on geologic scales” by which he confirms that, “gradualism may represent the most central conviction residing both within and behind all Darwin’s thoughts” (Gould, 2002). “Undirected” obviously reflects evolution’s non-purposeful, chance-driven outcomes. When populations produce “copious” potential solutions to environmental problems, then this affords a haphazard “hit and miss” approach to problem solving. The study of Astyanax CF and other troglobites provide models to test the accuracy of these contrasting predictions of how adaptation should be characterized.
In the case of Astyanax CF, at least 30 different cave populations have been documented as having multiple points of origin from the same surface morphotype and all the cave populations exhibit nearly the same morphological and behavioral traits (Herman et al., 2018; McGaugh et al., 2020b). Rather than invoking the empty term “convergent evolution,” these rapidly emerging and repeatable Astyanax CF variants are clearly consistent with an engineering-based model of adaptation that is inherently innate and purposeful. In this review on the subject of Astyanax CF, we not only show how exquisitely engineered the Astyanax CF adaptive remodeling system is, but the potential of this biological model for creationist research in the emerging field of continuous environmental tracking and adaptive engineering in living systems (Guliuzza and Gaskill, 2018; Hennigan and Guliuzza, 2019).
Morphological and Sensory Adaptations
The chief and most notable adaptation of interest in Astyanax CF compared to the surface fish (SF) morphotype is eye degeneration where the eyes are either extremely small and non-functional or nearly absent, and loss of pigmentation as shown in Figure 1 (Emam et al., 2020). This adaptation is especially intriguing because in contrast to most vertebrates, teleost fish have eyes that experience regenerative growth throughout their lives. The retina in teleosts is maintained throughout the life of a fish by a continuous acquisition of new cells from stem cell sources in two other parts of the eye structure (Otteson et al., 2001; Emam et al., 2020). However, in blind CF, the considerable resources that would otherwise be partitioned to the development and maintenance of the highly metabolic neural tissue of the eye are effectively partitioned to other necessary systems needed for dark adaptation.
During the initial stages of embryonic development, Astyanax CF eyes follow a similar pattern of development as SF, but after just a few hours, eye development arrests and the eyes degenerate through a controlled system of programmed cell death known as apoptosis (Krishnan and Rohner, 2017). This is no simple feat due to the fact that numerous genes are involved in eye development and many of these same genes also contribute to other aspects of development including the neural tissue of the brain. In other words, how is it that eye development is arrested while other types of neural development utilizing some of the same genes proceed either normally or are used to enhance troglobite-specific neural structures? The answer from an engineering-based approach would point to alternative developmental programs for dark versus light adaptation. In contrast, evolutionists have been flustered to some extent while investigating one candidate developmental gene after another because they are constantly looking for a single broken system component based on their Darwinian world-view. Nevertheless, important strides have been made in secular CF research despite handicaps that couple to the anti-design presuppositions that dominate biology.
Research guided by a theory of biological design would ask different questions. It would predict and search for a balancing mechanism that clearly must exist for both the timing and location of the expression of many developmental genes in the sighted vs. blind Astyanax morphotypes. As it turns out, the initial arresting of eye development observed in the blind CF is a necessary system constraint. It was at first assumed, due to the anti-design bias of the Darwinian framework, that the initial phase of normal eye development in blind Astyanax CF was a waste of the organism’s resources, but now it has become apparent that it is a requirement of neurodevelopment. The cells contributing to eye development and brain development are so highly intertwined that they must proceed in concert in the very beginning, but then the system takes a different logic-based pathway depending on whether it is heading towards a sighted or blind phenotype replete with a specified pathway of apoptosis. Programmed cell death (apoptosis) is a universal subsystem of embryo development across the spectrum of multicellular life.
Another major programmed modification of an extremely complex trait in Astyanax CF is the enhancement of the olfactory system (Blin et al., 2018; Jeffery, 2020; Sears et al., 2020). The Astyanax olfactory system is engineered to detect a variety of specified molecules related to foraging for food, communication with other fish, reproduction, and predator avoidance. The sensory organ is positioned in a multi-layered pit on the epidermis of the head. Each olfactory sensory neuron contains a single sensor (receptor) that detects a specific odorant representing only one of a wide array of specialized sensors. Compared to surface morphs, Astyanax CF have an olfactory system that is both physically larger and at least twice as sensitive to odorant molecule concentration. This conversion has been shown to be extremely rapid. Observations also support a model of a highly regulated and automated developmental process. For example, Astyanax SF embryos that have had one of their developing eye lenses ablated will develop a larger olfactory pit on the side of the head with the degenerate eye compared to the side with the normal eye (Yamamoto et al., 2003).
Yet another important Astyanax CF dark-adapted enhancement as a compensatory mechanism for vision loss involves a form of mechano-sensation through a series of specialized sensors on the skin that are collectively called the lateral line (Yoshizawa et al., 2010; Lloyd et al., 2018a; Maldonado et al., 2020). As in many different types of teleost fish, the lateral lines are comprised of individual sensor organs called neuromasts which are specialized hair cells. Neuromasts have stereocilia (hair-like structures) which project into the surrounding water from the skin and are found in two different sensor configurations. One form occurs as a single organ on the skin surface while the other is found in specialized canals. Both sensor configurations function by decoding signals generated from the perturbance of a gelatinous mix (cupula) that surrounds the base of each stereocilium. Each neuromast type is configured to be sensitive to a specified bandwidth of frequencies. The overall neuromast sensory system is able to detect and neurologically portray a complex hydrodynamic image of the surrounding aquatic environment in the brain of the fish (Tan et al., 2011; Yoshizawa et al., 2014; Yoffe et al., 2020). This accounts for aquarists’ observations of how store-bought blind Astyanax successfully navigate obstacles.
In research where the lateral line has been ablated, Astyanax exhibit impaired prey detection, loss of predator evasion, lack of obstacle avoidance, mating difficulties, and loss of water current detection (Yoshizawa et al., 2014; Yoffe et al., 2020). Compared to surface moprhs, Astyanax CF exhibit hypertrophy (enlargement) of the lateral line where neuromasts are longer and more numerous (Yoshizawa et al., 2014; Yoffe et al., 2020).
Another significant part of Astyanax cave adaptation that shows notable anatomical and physiological changes from surface vs. cave morphs is in the hypothalamus, a hormone producing organ located in the brain that is a principal regulator of homeostasis (Jaggard et al., 2018; Loomis et al., 2019; Maldonado et al., 2020). For the blind CF, these changes are a key part of the dark-adaptive phenotype influencing overall aggressiveness, sleep-wake cycles, and appetite. These programmed responses in the CF hypothalamus explain behavioral adaptations to darkness and other cave conditions. The levels of hypothalamic dopamine and serotonin in the brain are related to a variety of different adaptive CF behaviors (Elipot et al., 2013; Retaux and Elipot, 2013; Maldonado et al., 2020).
Metabolic and Physiological Adaptations
Interestingly, many of the Astyanax CF metabolic and physiological adaptations for dark-adapted cave life can appear quite rapidly while the full phenotype entailing the extreme developmental morphological adaptations seems to take longer, but also starts to develop quickly (see discussion below on “Cave Adaptation – How Fast?”). Researchers have recently documented the rapid emergence of CF metabolic and physiological traits in sighted surface fish raised in the dark for two years. They attribute the changes to phenotypic plasticity (Bilandzija et al., 2020). These dark adapted changes include the following traits: 1) overall decrease in metabolic rate associated with an increase in starvation resistance, 2) upregulation of fat metabolism genes with an increase in triglyceride levels, 3) increase in growth hormone levels, and 4) changes in pituitary and thyroid hormone levels (Bilandzija et al., 2020). Directly related to all of these system level metabolic effects are major alterations in gene expression related to hormonal signaling, signal transduction, protein metabolism, protein modification, numerous transcription factors, and genes involved in epigenetic modification of the genome (Gore et al., 2018; Bilandzija et al., 2020).
Behavioral Adaptations
Behavioral adaptations to perpetual life in a dark cave for Astyanax CF involve changes in sleep and circadian rhythms, social and group (schooling) activity, aggression, feeding, and stress-related activity (McGaugh et al., 2020a). Many of these alterations in behavior are connected to Astyanax CF phenotypic plasticity, adaptive deployments in morphology, and metabolism/physiology.
In regards to feeding, Astyanax SF forage their food from the surface or the upper layers of the water column, while CF target their food at the bottom or in the lower part of the water column (Borowsky, 2018). Astyanax CF also assume a different, more-horizontal, posture during feeding than surface fish which are nearly vertical in their posture (Jeffery, 2020). Many of the feeding differences in CF are facilitated by the mechano-sensory morphological adaptations in the lateral line of the fish discussed above.
Another lateral line-related behavioral shift in Astyanax compared to SF is the tendency of CF to swim toward the source of a water disturbance, whereas SF would disperse or flee. This is known as vibration attraction behavior (VAB) and is directly connected to the increased size and number of neuromasts in the head, but not to those of the lateral line (Yoshizawa et al., 2012; Fernandes et al., 2018). This behavior is advantageous for feeding and foraging in the dark. Research has shown that the VAB is directly connected to the mechanosensory function of the neuromasts and the specified sensitivity occurs within a frequency range of 10 to 50 Hz and peaking at 35 Hz (Yoshizawa et al., 2010). Furthermore, it has also been shown that VAB is unrelated to the auditory structure of Astyanax which is developmentally the same between CF and SF (Yoshizawa et al., 2010).
Further, CF were discovered to sleep considerably less than the surface morphs (Borowsky, 2018). In the laboratory on a 12-hour light–dark cycle, surface fish tend to sleep for 12 hours while cavefish sleep only three or four hours (Borowsky, 2018). This trait, only documented recently, may be almost universal in cavefishes, having been found in all five independent cave species populations where researchers have looked for it. Circadian rhythms are systems in an organism that not only interface with the solar day-night cycle, but help to regulate many aspects of physiology and behavior. Adaptation to a life in perpetual darkness involves an extreme change in this regard.
Overall aggressiveness is another important behavioral change to cave adaptation for Astyanax. Compared to surface morphs in the same regions where cave morphs exist, the CF are considerably less aggressive than SF and essentially shift their overall feeding behavior from fighting to foraging (Elipot et al., 2013; Retaux and Elipot, 2013) although at least one cave population was discovered where this was not the case (Espinasa et al., 2005). Nevertheless, the trait of non-aggression in Astyanax CF appears to be the norm and in developing embryos of SF where the eye was ablated, the adult fish still exhibited aggression indicating that the trait was non-eye related and a distinct part of the cave-adapted developmental program (Espinasa et al., 2005). The non-aggressive behavioral trait is likely linked to the hypothalamic changes discussed above and leads to improved efficiency for prey capture and finding food in caves, and has also been shown to improve foraging precision compared to SF (Lloyd et al., 2018a; Lloyd et al., 2018b). This trait is also related to schooling behavior as CF exhibit much less schooling than SF and tend to focus more on individual foraging (Kowalko et al., 2013).
In contrast to SF, the Astyanax CF have high appetites and engage in continuous feeding and foraging behavior to facilitate weight gain and fat deposition (especially during periods of food availability) that can be used later during periods of famine (Jeffery, 2020). In contrast to other vertebrates (e.g., humans), the excessive fat accumulation in the enlarged livers of the CF poses no obvious threat as no differences in the health or lifespan have been found compared to SF. In addition, the CF also have lower metabolic rates and increased resistance to weight loss that help them to survive during periods of low food availability (Aspiras et al., 2015).
Genetic Studies Don’t Support the Darwinian Model
Evolutionist CF researchers have searched repeatedly for some sort of mutation that could be interpreted within their Darwinian model as being magically selected by a cave environment to bring forth the Astyanax CF phenotype. This hope was recently reiterated in a review on the subject where the authors state, “Another important goal in the field of evolutionary genetics is to trace the origins of mutations necessary for adaptation” (Krishnan and Rohner, 2017). The authors then go on to expound upon the mutation-selection quandary stating, “Do novel mutations originate in the new environment (in this case, the cave) or do they already exist at some low frequency in the founder populations (the surface fish)?” and then the authors go on to speculate as to whether the alleged natural selection for the CF phenotype is direct, indirect, or not at all (neutral model-based evolution). Finally, they have to conclude from the genetic data that there is some complex multigenic adaptive system defying their paradigm, stating, “The strongest genetic argument for the hypothesis that eye loss is adaptive comes from QTL experiments.”
So what are QTL experiments and how have they defied evolutionary expectations? The term QTL stands for quantitative trait loci. Given that Astyanax CF and SF are nearly identical genetically and readily interbreed, researchers have made crosses and then analyzed the segregating progeny with molecular markers – mapping their genomic locations with various traits related to the CF phenotype. They are QTL because the diversity of traits that make up the CF phenotype are controlled by numerous genes and regulatory elements across the genome. In the statistical output from such studies, certain genetic markers that are significantly associated with a trait (e.g., eye size), will contribute a statistically significant amount of variability to the trait in question. In such a scenario, a major QTL might explain up to 30% of the overall trait variability.
Yet another line of studies performed in a separate field of genetics further discredits the long-standing evolutionary notion that discrete mutational effects underlie Astyanax CF adaptation. Several studies have sought to ascertain genetic diversity and the possibility of gene flow (interbreeding) between surface and blind cave populations (Bradic et al., 2012; Herman et al., 2018). The earlier study in 2012 used molecular markers for 11 cave and 10 surface populations and, as expected, found greater diversity among the larger surface populations and less diversity among the cave populations. However, they also discovered a small but significant amount of interbreeding between CF and SF, but nothing directly pointed to a genetic basis for the CF phenotype. How could such different morphotypes not also carry equally extreme mutational differences? The later study in 2018 actually sequenced the genomes of 47 Astyanax from three cave populations, two surface populations, and several outgroups. While this study did little to add to the previous one, it did show that while various CF genomes in different cave systems had unique points of origin based on separate colonization events, the Astyanax genomes were overall very similar and nothing distinctly related to conferring the blind CF phenotype could be pinned down.
In contrast to the evolutionarily unproductive nature of QTL mapping and genome sequencing studies, research in epigenetic modifications undergirding cave adaptation in Astyanax has been very fruitful, but not in favor of the Darwinian mutation-selection story. In fact, in a recent groundbreaking epigenetic study on Astyanax CF, the authors note the Darwinian quandary in their introduction, stating, “recent sequencing of the Pachón cavefish genome and other studies revealed no inactivating null mutations in essential eye development genes” (Gore et al., 2018). In this study, researchers investigated a type of epigenetic modification called cytosine methylation where specified regulatory sections of the genome in and around genes will have a methyl group attached to a cytosine in the genetic code. This site-directed methylation effectively down-regulates or suppresses certain types of gene activity. The research showed that DNA methylation-based epigenetic silencing was an adaptive mechanism in Astyanax CF eye degeneration. By performing parallel analyses in both cave and surface morphs, and also in the zebrafish (Danio rerio) as another sighted fish, they discovered that DNA methylation confers eye-specific gene repression and also regulates early eye development. These epigenetic data confirm the fact that the overall blind CF phenotype is an inherent system-wide, pre-programmed, adaptive response.
Cave Adaptation – How Fast?
At what speed does dark-adaptive phenotypic plasticity develop in Astyanax? As noted above, metabolic and physiological changes found in blind cavefish can be found within a single generation of dark-raised surface Astyanax (Bilandzija et al., 2020). Furthermore, the beginning of morphological changes can also be observed within a single generation of dark-raised surface fish in only a two-year period (Bilandzija et al., 2020). Such immediate changes include an increase in overall retinal thickness of the eyes, thinning of plexiform layers (optical areas of neuronal connection), and specifically a thickening of the nuclear and photoreceptor layers of the retina. The overall body shape of the fish also changed.
In regards to how long the full blind CF morphotype takes to form, the jury is still out on the matter, although evolutionary ideas are quickly being upended in rapid succession. Original Darwinian hypotheses that frame adaptation as a gradual, haphazard or “hit and miss” process estimated the divergence of blind Astyanax morphotypes from sighted surface populations at about 3.1 to 8.1 million years ago (Strecker et al., 2004; Ornelas-Garcia et al., 2008). Then about a decade later, several more evolutionary studies came up with much more recent deep-time estimates with one study arriving at a divergence time of about 115,000 to 190,000 years ago (Herman et al., 2018) while another study published about the same time yielded an estimate of 20,000 to 100,000 years ago (Fumey et al., 2018). Clearly, something was amiss in these evolutionary studies that all produced wildly different deep-time estimates.
In 2020, a groundbreaking study was published showing that a recently established population of sighted surface Astyanax in a Texas stream had invaded a nearby cave aquifer and had morphed into a blind CF phenotype in less than 100 years (McGaugh et al., 2020b). The authors of this study state, “our results suggest that morphological and behavioural changes accompanying cave colonization can be established rapidly, and this system offers an exciting and unique opportunity for isolating the genetic and environmental contributions to colonization of extreme environments.” The hypothetical deep-time evolutionary models can now be cast aside in light of this recent very pragmatic data. We now have direct evidence that the Astyanax CF morphotype can develop within less than 100 hundred years and that as discussed previously, the beginnings of morphological, metabolic, and physiological dark-adapted traits can develop in less than one generation of dark-reared SF Astyanax (Bilandzija et al., 2020).
Summary
So why or how would fish end up living in a cave to begin with? It is possible they could have been swept in through a flood or they may have simply colonized the environment with their innate creature abilities as part of the process of obeying the God-given mandate to fill the earth and its ecological niches as engineered by the Creator. Mixes of surface and blind cave Astyanax have been found in intermediate zones near the entrances of cave systems along with intermediate morphotypes in the population (Bradic et al., 2012). Furthermore, cave environments do provide some advantages such as a refuge from intense predation, decreased competition with other fish, and a shelter from weather extremes.
In a recent review on Astyanax CF, the authors state, “Since the publication of Darwin's Origin of Species, cave animals have been subjects of conjecture about the forces driving evolution” (McGaugh et al., 2020a). But have these cave animals, and especially the well-researched Astyanax CF, revealed any evidence of Darwinian selectionism or do the data point to exquisitely engineered systems that track their environments and subsequently deploy a complete remodeling of the organism to both adapt and strategically position itself to succeed? The data increasingly favor the latter. This trend confirms what Guliuzza (2012) had proposed—that an engineering-based CET model of adaptation would make accurate predictions towards the study of adaptation, facilitating the identification of innate mechanisms controlling the development of cave-specific traits.
Given that Astyanax CF have been well-researched in the secular community and are relatively easily studied, reared, and bred in a laboratory setting, we propose using these fish in creationist research for the study of adaptive engineering and environmental tracking in conferring phenotypic plasticity in adaptation.
References
Aspiras, A.C., N. Rohner, B. Martineau, R.L. Borowskyand, and C.J. Tabin. 2015. Melanocortin 4 receptor mutations contribute to the adaptation of cavefish to nutrient-poor conditions. The Proceedings of the National Academy of Sciences, U.S.A. 112(31): 9668-9673.
Bilandzija, H., B. Hollifield, M. Steck, G. Meng, M. Ng, A.D. Koch, R. Gracan, H. Cetkovic, M.L. Porter, K.J. Rennerand, and W. Jeffery. 2020. Phenotypic plasticity as a mechanism of cave colonization and adaptation. eLife 9: e51830. doi: 10.7554/eLife.51830.
Blin, M., E. Tine, L. Meister, Y. Elipot, J. Bibliowicz, L. Espinasaand S. Retaux. 2018. Developmental evolution and developmental plasticity of the olfactory epithelium and olfactory skills in Mexican cavefish. Developmental Biology 441(2): 242-251.
Borowsky, R. 2018. Cavefishes. Current Biology 28(2): R60-R64.
Bradic, M., P. Beerli, F.J. Garcia-de Leon, S. Esquivel-Bobadillaand, and R.L. Borowsky. 2012. Gene flow and population structure in the Mexican blind cavefish complex (Astyanax mexicanus). BMC Evolutionary Biology 12: 9 doi: 10.1186/1471-2148-12-9.
Elipot, Y., H. Hinaux, J. Callebertand, and S. Retaux. 2013. Evolutionary shift from fighting to foraging in blind cavefish through changes in the serotonin network. Current Biology 23(1): 1-10.
Emam, A., M. Yoffe, H. Cardonaand, and D. Soares. 2020. Retinal morphology in Astyanax mexicanus during eye degeneration. The Journal of Comparative Neurology 528(9): 1523-1534.
Espinasa, L., Y. Yamamotoand, and W.R. Jeffery. 2005. Non-optical releasers for aggressive behavior in blind and blinded Astyanax (teleostei, characidae). Behavioural Processes 70(2): 144-148.
Fernandes, V.F.L., C. Macaspac, L. Luand, and M. Yoshizawa. 2018. Evolution of the developmental plasticity and a coupling between left mechanosensory neuromasts and an adaptive foraging behavior. Developmental Biology 441(2): 262-271.
Fumey, J., H. Hinaux, C. Noirot, C. Thermes, S. Retauxand, and D. Casane. 2018. Evidence for late Pleistocene origin of Astyanax mexicanus cavefish. BMC Evolutionary Biology 18(1): 43.
Gore, A.V., K.A. Tomins, J. Iben, L. Ma, D. Castranova, A.E. Davis, A. Parkhurst, W.R. Jeffery, and B.M. Weinstein. 2018. An epigenetic mechanism for cavefish eye degeneration. Nature Ecology and Evolution 2(7): 1155-1160.
Gould, S.J. 2002. The Structure of Evolutionary Theory. Cambridge, MA: Harvard University Press, pp. 141-148.
Gross, J.B. 2012. The complex origin of Astyanax cavefish. BMC Evolutionary Biology 12(1): 105.
Guliuzza, R.J. 2012. Engineered adaptability. Acts & Facts 41(10): 11-14.
Guliuzza, R.J., and P.B. Gaskill. 2018. Continuous environmental tracking: An engineering framework to understand adaptation and diversification. In Proceedings of the Eighth International Conference on Creationism, (ed. J.H. Whitmore), pp. 158-184. Creation Science Fellowship, Pittsburgh, PA.
Hennigan, T. and R. Guliuzza. 2019. The continuous environmental tracking hypothesis—application in seed dormancy and germination in forest ecosystems. Journal of Creation 33(2): 77-83.
Herman, A., Y. Brandvain, J. Weagley, W.R. Jeffery, A.C. Keene, T.J.Y. Kono, H. Bilandzija, R. Borowsky, L. Espinasa, K. O'Quin, C.P. Ornelas-Garcia, M. Yoshizawa, B. Carlson, E. Maldonado, J.B. Gross, R.A. Cartwright, N. Rohner, W.C. Warrenand S.E. McGaugh. 2018. The role of gene flow in rapid and repeated evolution of cave-related traits in Mexican tetra, Astyanax mexicanus. Molecular Ecology 27(22): 4397-4416.
Jaggard, J.B., B.A. Stahl, E. Lloyd, D.A. Prober, E.R. Duboueand A.C. Keene. 2018. Hypocretin underlies the evolution of sleep loss in the mexican cavefish. eLife 7: e32637 doi: 10.7554/eLife.32637.
Jeffery, W.R. 2020. Astyanax surface and cave fish morphs. Evolutionary Developmental Biology 11: 14. doi: 10.1186/s13227-020-00159-6.
Kowalko, J.E., N. Rohner, S.B. Rompani, B.K. Peterson, T.A. Linden, M. Yoshizawa, E.H. Kay, J. Weber, H.E. Hoekstra, W.R. Jeffery, R. Borowskyand, and C.J. Tabin. 2013. Loss of schooling behavior in cavefish through sight-dependent and sight-independent mechanisms. Current Biology 23(19): 1874-1883.
Krishnan, J., and N. Rohner. 2017. Cavefish and the basis for eye loss. Philosophical Transactions of the Royal Society of London B Biological Sciences 372(1713) : 20150487.
doi: 10.1098/rstb.2015.0487.
Lloyd, E., C. Olive, B.A. Stahl, J.B. Jaggard, P. Amaral, E.R. Duboueand, and A.C. Keene. 2018a. Evolutionary shift towards lateral line dependent prey capture behavior in the blind Mexican cavefish. Developmental Biology 441(2): 328-337.
Lloyd, E., C. Olive, B.A. Stahl, J.B. Jaggard, P. Amaral, E.R. Duboueand, and A.C. Keene. 2018b. Evolutionary shift towards lateral line dependent prey capture behavior in the blind Mexican cavefish. Developmental Biology 441(2): 328-337.
Loomis, C., R. Peuss, J.B. Jaggard, Y. Wang, S.A. McKinney, S.C. Raftopoulos, A. Raftopoulos, D. Whu, M. Green, S.E. McGaugh, N. Rohner, A.C. Keeneand, and E.R. Duboue. 2019. An adult brain atlas reveals broad neuroanatomical changes in independently evolved populations of Mexican cavefish. Frontiers in Neuroanatomy 13: 88. doi: 10.3389/fnana.2019.00088.
McGaugh, S.E., J.E. Kowalko, E. Duboue, P. Lewis, T.A. Franz-Odendaal, N. Rohner, J.B. Grossand, and A.C. Keene. 2020a. Dark world rises: The emergence of cavefish as a model for the study of evolution, development, behavior, and disease. Journal Experimental Zoology B 334(7-8): 397-404.
McGaugh, S.E., S. Weaver, E.N. Gilbertson, B. Garrett, M.L. Rudeen, S. Grieb, J. Roberts, A. Donny, P. Marchettoand, and A.G. Gluesenkamp. 2020b. Evidence for rapid phenotypic and behavioural shifts in a recently established cavefish population. Biological Journal of the Linnean Society 129(1): 143-161.
Maldonado, E., E. Rangel-Huerta, E. Rodriguez-Salazar, E. Pereida-Jaramilloand A. Martinez-Torres. 2020. Subterranean life: Behavior, metabolic, and some other adaptations of Astyanax cavefish. Journal Experimental Zoology B 334(7-8): 463-473.
Ornelas-Garcia, C.P., O. Dominguez-Dominguez, and I. Doadrio. 2008. Evolutionary history of the fish genus Astyanax baird & girard (1854) (Actinopterygii, Characidae) in Mesoamerica reveals multiple morphological homoplasies. BMC Evolutionary Biology 8(1): 340.
Otteson, D.C., A.R. D'Costaand, and P.F. Hitchcock. 2001. Putative stem cells and the lineage of rod photoreceptors in the mature retina of the goldfish. Developmental Biology 232(1): 62-76.
Retaux, S., and Y. Elipot. 2013. Feed or fight: A behavioral shift in blind cavefish. Communicative & Integrative Biology 6(2): e23166.
Sears, C.R., T.E. Boggsand, and J.B. Gross. 2020. Dark-rearing uncovers novel gene expression patterns in an obligate cave-dwelling fish. Journal of Experimental Zoology Part B: Molecular and Developmental Evolution 334(7-8): 518-529.
Strecker, U., V.H. Faundezand, and H. Wilkens. 2004. Phylogeography of surface and cave Astyanax (Teleostei) from Central and North America based on cytochrome b sequence data. Molecular Phylogenetics and Evolution 33(2): 469-481.
Tan, D., P. Pattonand, and S. Coombs. 2011. Do blind cavefish have behavioral specializations for active flow-sensing? Journal of Comparative Physiology A 197(7): 743-754.
Yamamoto, Y., L. Espinasa, D.W. Stockand, and W.R. Jeffery. 2003. Development and evolution of craniofacial patterning is mediated by eye-dependent and -independent processes in the cavefish Astyanax. Evolutionary Development 5(5): 435-446.
Yoffe, M., K. Patel, E. Palia, S. Kolawole, A. Streets, G. Haspeland, and D. Soares. 2020. Morphological malleability of the lateral line allows for surface fish (Astyanax mexicanus) adaptation to cave environments. Journal Experimental Zoology Part B: Molecular and Developmental Biology 334(7-8): 511-517.
Yoshizawa, M., S. Goricki, D. Soaresand, and W.R. Jeffery. 2010. Evolution of a behavioral shift mediated by superficial neuromasts helps cavefish find food in darkness. Current Biology 20(18): 1631-1636.
Yoshizawa, M., W.R. Jeffery, S.M. van Nettenand, and M.J. McHenry. 2014. The sensitivity of lateral line receptors and their role in the behavior of Mexican blind cavefish (Astyanax mexicanus). Journal of Experimental Biology 217(Part. 6): 886-895.
Yoshizawa, M., Y. Yamamoto, K.E. O'Quinand, and W.R. Jeffery. 2012. Evolution of an adaptive behavior and its sensory receptors promotes eye regression in blind cavefish. BMC Biology 10: 108.