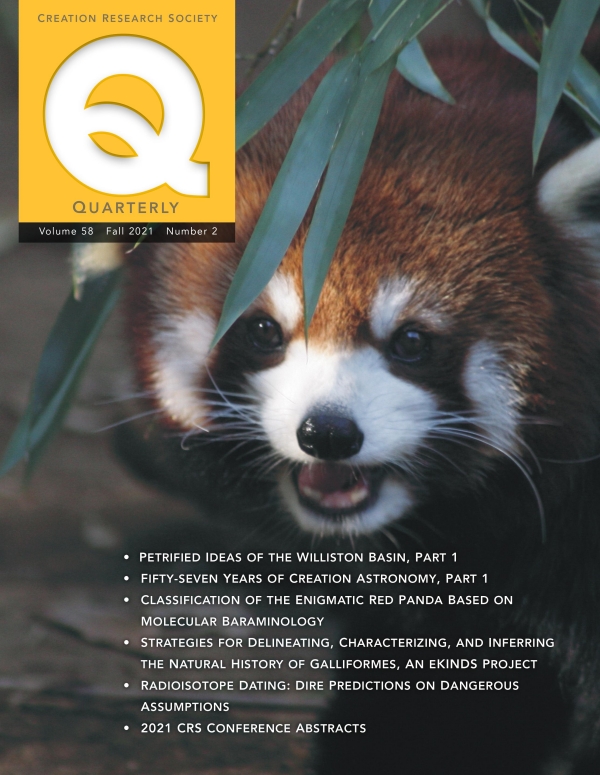
ABSTRACTS
The Williston Basin of central North America is famous for its “nearly complete geologic column,” and its huge fossil fuel resources. As an incratonic basin with relatively few unconformities and representatives of each of the purported geologic time periods, it has been a poster child for deep time. However, many features appear at variance with uniformitarianism and an ancient Earth. These include the thick sedimentary sequence, gravel-capped planation surfaces, petrified wood and other fossils, and fossil fuels. The geologic setting is presented, showing weaknesses and strengths in the diluvial hypothesis of the basin’s origin and history. The mental straitjacket of uniformitarianism results in petrified ideas that hinder understanding of likely geologic processes. This is particularly demonstrated by extensive planation surfaces with gravel veneers of largely exotic lithologies, which are readily explained by diluvial processes but not by uniformitarianism.
Introduction
The Williston Basin is one of the “big four shale oil” plays at the heart of the U.S.A.’s revival in oil production that has virtually eliminated American dependence on foreign oil (Figure 1). But the Williston Basin is famous for other reasons. It contains a huge accumulation of sedimentary rocks that purport to show a nearly-complete stratigraphic sequence (Fowler and Nisbet, 1985; Morton, 2001), challenging advocates of a young earth and global Flood (i.e. diluvialists). But not so fast! Evolutionists have problems there, too. The confusion between science and natural history (Reed and Klevberg, 2017, 2018)—especially conflating a priori speculation with data—has hindered stratigraphy, but recent decades have seen a progressively more scientific (albeit uniformitarian) stratigraphic approach in the Williston Basin. As in many parts of the world, the area encompassed by the Williston Basin also exhibits planation surfaces that provide strong evidence for the Deluge. In this paper, we will discuss a few of these issues, and we will provide some geologic background for the examples of fossilization in the Fort Union Group that will be the theme of Part II, which will appear in a subsequent issue
The Williston Basin
The basin is an oval shaped depression (Figure 2) filled with up to 5,000 meters of sedimentary rocks. Limestones and dolostones are more common in the deeper “Paleozoic” strata, and clastics are more common in shallower “Cenozoic” strata, but there is much alternation and variation, and salts ("evaporites") also occur and act as top seals for petroleum systems. Rich petroleum plays have resulted in a wealth of borehole (subcrop) and geophysical data. Our area of study is between Watford City and Medora, western North Dakota, in the southern part of the basin (Figure 3). This covers most of the famous badlands area of the state.
Structure
The Williston Basin is widely believed to be a cratonic or intracontinental basin that gradually sank at a rate constant enough for almost continuous accumulation of sediments, representing the entire geologic column from the Cambrian to the Cenozoic (Morton, 2001). Minor Laramide folding and faulting, dated latest Cretaceous to early Tertiary in the evolution-based age scheme, is present (Hamdani et al., 1994). The center of the basin is just southeast of Watford City (Figure 4), and the depth to basement there is approximately 5,000 m (16,350 ft) from the present land surface (Anderson, 2009). Important structural features are shown on Figure 2.
Stratigraphy
While all the “periods” of the geologic column are commonly believed to be present in the Williston Basin, several unconformities are also acknowledged. Some of the most pronounced are indicated by wiggly contact lines on Figure 5. A stratigraphic column is shown on Figure 6. Some unconformities occur near the edges of the basin but transition into conformities in the center (LeFever, 1991). This supports the belief that Williston Basin unconformities represent sequence boundaries (and subsequence boundaries) following the current sequence stratigraphic paradigm, interpretations of which can conflict with inferred subsidence history seen through uniformitarian-colored glasses (e.g. Zhu and Hajnal, 1993; Sonnenberg, 2017).
One might think the Williston Basin would provide an unusually simple exercise in stratigraphy. But it is more complex. It is well known that efforts at radiometric dating are very much “after the fact” (i.e. chronostratigraphic) and generally forced to conform to "known" dates (Oard, 2019). This is doubly the case in a sedimentary basin such as the Williston Basin, since the sedimentary rocks are ill suited for radiometric dating (most methods are limited to igneous rocks). Most dates were assigned well prior to radiometric methods and were based on biostratigraphy, i.e index fossil dating. It is almost impossible to read any of the early papers on the area without finding references to flora and fauna of particular ages being used to pigeonhole various strata into the evolutionary time scheme.
Scientific progress has been made, even with the deleterious effect of evolutionary bias on the part of most geologists. Whereas early work often focused on age-classifying formations based on the assumption of evolution, modern work has focused more on unconformity-bounded units with evolution only implicit in the work. Thus, there has been a shift from age-inferred classification to physically-based criteria. We will particularly address two areas of progress: the basin as a whole, and the uppermost Fort Union strata (Figure 6) that are the focus for much of this series.
If radiometric dating is limited and evolutionary fossil succession fails to deliver its touted means of deciphering the rocks, then geologists must rely on other means—often on scientific means—to delineate rock units. Lithostratigraphy, which correlates rocks based on their physical properties, is one method. This was complicated by "facies model" analysis, popular in the late 20th century, and recognized by the application of "Walther's Law" to strata (see glossary). A highly successful scientific technique is seismic measurements to produce two- and three-dimensional imaging of subsurface layering and structure. This geophysical method facilitates mapping of subsurface features that previously had to be inferred between wells across the basin.
Seismic data also propagated new ideas in stratigraphy. While there were many notable participants, the man in the middle was Larry Sloss. From 1937 to 1947 he taught at the lead author’s alma mater in Butte, Montana, and worked for the Montana Bureau of Mines and Geology doing a good deal of field work during the summers (Dott, 2014). Sloss traced “. . . several important unconformities in the Montana region, which did not conform to any classical, fossil-based stratigraphic divisions . . .” (Dott, 2014, p. 24) to North Dakota, which sparked ideas that he later developed with his colleagues at Northwestern University. Later students and successors (most famously the “Exxon Group”) carried the concepts further to create sequence stratigraphy, which has become the predominant interpretive framework in sedimentary geology well beyond the oil patch.
Even as evolutionists, these pioneering stratigraphers recognized the tension between evolution and the data of science. “By the 1930s, Laurence L. Sloss noted that stratigraphy was beset with 'stupefying arguments' about abstract time versus actual rocks and how to classify such things” (Dott, 2014, p. 24). While not without uniformitarian baggage (Klevberg, 1999; 2000a; 2000b), sequence stratigraphy has been a leap in an empirical direction, a fact that garnered resistance from many establishment geologists. The Williston Basin is now almost universally mapped using sequence stratigraphic interpretations, though that entails the necessity of a fine equilibrium of gradually rising and falling sea levels (eustasy) over hundreds of millions of years. Several researchers have warned about the difficulty if not impossibility of correlating cratonic sequences (a.k.a. supersequence, sloss sequence, megasequence) to other continents, showing the effort has met with only limited success, and asserting that eustasy is unlikely to be the sole responsible mechanism (Christie-Blick and Driscoll, 1995; Wilson, 1998; Bailey and Smith, 2010; Catuneanu et al., 2011). Some have argued from logic and experimental evidence that such correlation can only provide nonunique solutions (Milana and Tietze, 2007). Despite this lack of correspondence outside the sedimentary basin, Clarey and Werner have used sequence stratigraphic principles to attempt a global correlation and revised eustatic curve that they assert shows a diluvial signature (Clarey and Werner, 2017; 2020). The validity of the sequence stratigraphic approach to correlation is dependent on the validity of the assumption of eustasy, which is arguably easier to support on the basis of the historic Deluge than speculated fluctuations over great ages. This stratigraphic revolution began in Montana and North Dakota and the Williston Basin, and so we will examine the basin as diluvialists, particularly the Fort Union Group (Figures 5 and 6).
Fort Union Group
Fort Union strata were some of the earliest units in the Williston Basin to be studied, as they provide outcrops for study at the surface. They host the fossil wood that will be the focus of Part II and the low-rank coal (lignite) that will be addressed in Part III. Formation of badlands topography that cuts into four (possibly five) of these formations provides many outcrops for direct study. One might think the stratigraphic classification of well-exposed, undeformed strata would have been unusually simple, but not so. There has been much "evolution" of stratigraphic nomenclature over time (Figure 7), not only due to increases in data, but also from changes in interpretive frameworks.
Some of the reasons for changes in terminology are illustrated by the Ludlow Formation at the base of the Fort Union Group:
The beds now placed in the Ludlow Formation have been variously referred to as the Laramie, Lance, and Fort Union ‛Formations’ and thus assigned a Cretaceous, Paleocene, or Eocene age. This complexity of nomenclature and age assignment arose because of the gross similarity of the rocks above the marine Cretaceous. This thick, monotonous sequence required a long period of study over a large area by many workers before criteria were established which effectively subdivided the strata according to color and lignite content.... (Moore, 1976, p. 11).
Biostratigraphy was thereby succeeded by the more empirical lithostratigraphy. Starting with the original type section in extreme eastern Montana (Figure 8), various members were identified and mapped. The type section for the Fort Union Formation was moved from Fort Union to near Livingston, Montana, far to the southwest, which is now the section recognized by the U.S. Geological Survey. Exposures along the Tongue River in southeastern Montana and northeastern Wyoming at the north end of the Powder River Basin (Figure 2) gave the name to the Tongue River Member or Tongue River Formation. Clayton et al. (1977) decrying the fact that nothing but an interpreted age directly linked the Tongue River exposures to the rocks observed around Medora, North Dakota, established new terminology for the Fort Union Group and a new type section for the Bullion Creek Formation in North Dakota (Clayton et al., 1977). Strata still referred to by the U.S. Geological Survey as Tongue River Member on the Montana side of the border along the Yellowstone River are Bullion Creek Formation in Klevberg’s experience. We think this is an example of evolutionists who are good scientists and who allow the geologic classification to be constrained by physical evidence.
With experience, it is possible to recognize the Bullion Creek and Sentinel Butte Formations as separate formations. The two have mineralogic differences (see Table I) and visible differences in color and weathering patterns (Figure 9). Igneous minerals are common in these formations, including much bentonite, volcanic ash altered in sea water. However, there are also metamorphic minerals and igneous rock fragments that indicate source areas in the Black Hills or Rocky Mountains, a great distance from North Dakota (Jacob, 1975). This is especially problematic for believers in deep time since mica and some other minerals found in the Fort Union Group would tend to weather rapidly and thus must have been transported quickly and deposited immediately. Fossils, both animal and plant, are typically in distinct horizons or isolated pockets only (Jacob, 1977; Biek and Gonzalez, 2001). Lignite beds are common in both formations.
While lignite beds occur throughout the Fort Union strata, petrified wood is not as widely distributed. In Klevberg’s experience, while petrified wood is abundant, it appears limited to a few horizons, primarily near the base (Figure 10). This has been observed throughout the area (Biek and Gonzalez, 2001). In our experience, it occurs primarily in bentonitic strata at the base of the Sentinel Butte Formation, but it sometimes appears associated with lignite strata higher up in the formation. The specimen shown in the lower part of Figure 11 was observed part way up the lower units of the Sentinel Butte Formation approximately 68 km north-northeast of the “Petrified Forest” featured in Part II of this series. The lignite from which this specimen originated has burned at this locality to form clinker. Clinker is very apparent in the area due to the bright orange or red color commonly formed as burning lignite has been observed to bake overlying clay into "natural brick" (Biek and Gonzalez, 2001). It is possible that the larger mass of wood from which the specimen in Figure 11 formed was not petrified but only coalified and hence burned with the lignite seam. Partially petrified/coalified wood has been observed elsewhere (Williams, 1996) and appears to demand rapid chemical alteration to prevent normal decay. Biek and Gonzalez (2001) also noted silicified lignite, a combination of coalification and silicification as opposed to the either/or sample Williams (1996) wrote about (Biek and Gonzalez, 2001).
The “Petrified Forest” occupies a slightly lower elevation than the Big Plateau as indicated by the fine contours on the geologic map in Figure 12. The petrified wood horizons are extensive and can be traced in the field east across the Little Missouri River and clear across the south unit of Theodore Roosevelt National Park, as well as north 70 km to the north unit of the park, where horizons proliferate higher up in the formation (Biek and Gonzalez, 2001). Lignite horizons of various thicknesses are evident throughout, and the thickest are sometimes useful as marker beds. While some major beds can be traced for several miles or more, most are not so laterally extensive, and a minor bed observed in one locality may not be the same as one in a similar position elsewhere.
Geomorphology
Western North Dakota is characterized by gently rolling plains, fiercely dissected by a characteristic erosional topography known as “ badlands” and sprinkled with buttes formed of various formations that post-date the Fort Union Group. Vestiges of planation surfaces are not hard to find, and these are often capped by a coarse gravel veneer. Relict channels near the inferred extent of glaciation tend to be smooth, broad, and shallow, contrasting with the modern valley of the Little Missouri River.
Problems for Diluvialists
In some respects, the Williston Basin has been a poster child for traditional explanations and a daunting challenge to diluvialists. The Williston Basin is supposed to present a nearly complete example of the geologic column. While various fossils are present, fossil wood is prominent, especially in the Fort Union strata (marine microfossils are more prevalent deep in the basin). As we will develop in the next paper, fossil wood is widely presented as a "petrified forest" representing the passage of significant time.
Formation of the Williston Basin
We do not know how the Williston Basin formed. With no written record to rely on, there are only speculations and varying infusions of imagination in the stories proferred for its formation, ideally tempered with observations from the present. Was it an antediluvian feature, or did it form early in the Deluge? Oard (2013a) favors a bolide impact. Subsurface data have been interpreted as indicating an infilled impact crater beneath Red Wing Creek southwest of Watford City, North Dakota (Red Wing Creek Valley indicated by lighter colored stripe on Figure 4), but that is within the basin sediments. More than 1,800 m of strata had already been deposited when this impact occurred (Biek and Gonzalez, 2001), indicating it would have happened during the Genesis Flood and affected a very limited part of the Williston Basin. Tectonic means of subsidence have been proposed, possibly linked to the fountains of the great deep and the Mid Continent Rift (Clarey, 2020). Still other possibilities include mineral phase changes and crustal cooling.
Preposterously fast sedimentation?
The Williston Basin (Figure 2) contains nearly 5,000 m (more than 16,000 ft.) of sedimentary rocks that are believed to have accumulated in a gradually subsiding basin over much of earth history and thus provides an example of a rock column that illustrates a nearly complete geologic column (Peterson, 1988). It could be that a total of more than three times that thickness was deposited in the Williston and surrounding depositional basins (Peterson, 1988). Both the volume and inferred geologic column have been used, like the lower Mississippi Valley (Hayward, 1985), as an argument by anti-creationists (Morton, 2001).
To make matters worse for creationists, time available for deposition would have been less than a year. Deposition probably waned and widespread erosion commenced somewhere around Day 270 of the Deluge (Oard and Reed, 2017), leaving less than a year for deposition. The rate of deposition and the volume of sediments to fill the Williston Basin dwarf anything occuring today. As long as belief in uniformitarianism or actualism discouraged research in actual sedimentation processes, the anti-creationist argument from sediment volume appeared compelling (Reed and Oard, 2017). That began to change with progress in hydrodynamics and sedimentation processes.
To fill the Williston Basin in 270 days would require a minimum of 19 m/day (62 ft/day) on average. To supply the maximum inferred basin fill thickness for the Williston and surrounding basins in that time would require approximately 60 m/day of average sediment supply (depending on how one estimates or models total thickness and compression during lithification). Is a rate between 19 m/day and 60 m/day reasonable in light of empirical knowledge of sedimentation? In applying empirical equations for sand transport and deposition to the Hawkesbury Sandstone of Australia, Allen (1996) estimated that this extensive sandstone body likely was deposited at a rate of at least 62 m/day. Barnhart (2011) studied splay deposits in New Orleans formed from a levee breach during Hurricane Katrina for which time constraints indicated deposition occurred much more rapidly than 2.3 m/hr (equal to 55 m/day) and probably closer to 43 m/hr (approximately 1,000 m/day). Applying empirical relations to the Tapeats Sandstone of the Grand Canyon of the Colorado River, Barnhart (2012a; 2012b) estimated a rate for the basal Tapeats equivalent to approximately 215-1,300 m/day and for the middle and upper Tapeats equivalent to approximately 360 m/day. While a much more detailed analysis of Williston Basin infill is certainly warranted, these findings make it clear that filling the basin during the Flood year described in Genesis is realistic to the extent one can extrapolate from smaller scale geologic events. In fact, it is necessary for the sediments to have been deposited relatively rapidly based on these empirical relationships. Thus, for those who believe in "deep time,” nearly all of the purported millions of years of time were "dead time" in which no net deposition occurred, since the strata must be riddled with time gaps to accommodate the supposed ages of deposition, and most of these are “invisible” and not easily accommodated by recognized unconformities. While some unconformities are inferred (Figure 5), trying to explain this much "dead time" is clearly problematic for uniformitarians (Reed, 2002a,b; 2004).
Despite the fact that the sedimentation problem fits on the other foot, the idea of slow filling of a gradually subsiding basin persists and provides faulty ammunition for anti-creationists. Arguments of anti-creationist Hayward (1985) for equilibrium based on isostasy throughout central North America are readily and frequently refuted by old-earth-believing geologists themselves as attested by the various competing models for formation of the Williston Basin (Haid, 1991). Hayward’s argument for lack of accommodation space is also a straw man argument and refuted by the possibility of bolide impacts, lithospheric cooling, mineral phase changes, or other processes that create accommodation space without faulting.
Generating oil and coal
The Fort Union Group contains immense amounts of low-rank coal. Deeper Williston Basin formations contain enormous amounts of oil. We acknowledge this is an important argument of anti-creationists. Because the origin of fossil fuels deserves to be addressed at greater length than can be accommodated here, it will be the topic of Part III of this series.
Locating the D/P boundary
The geologic boundary marking the end of the Genesis Flood, the "Flood/post-Flood" or "diluvial/postdiluvial" (D/P) boundary, has been an object of intense discussion by creationists (Oard, 2014; Clarey and Werner, 2019). Clarey has argued for a higher D/P boundary than many previous researchers, some of whom were enamored with the legendary K/T (or K/Pg) boundary (Clarey, 2017). One of several important criteria for located the D/P boundary is geomorphology (Oard, 2018), especially planation surfaces. Planation surfaces are inimical to uniformitarian processes and a clear indication of highly energetic sheet flow (Oard, 2018). While the planation surfaces are not as dramatic in highly eroded western North Dakota as some other parts of the world, erosional remnants are preserved in Fort Union and overlying strata. In addition, the Bullion Creek and Sentinel Butte Formations (Figure 6) comprise approximately 425 m (1,400 ft.) of continuous, rapid deposition completely covering the Williston Basin and surrounding areas, which is strong evidence that the D/P boundary is higher in the section. Thus, accounting for the Fort Union Group and the coal and fossil wood it contains after the Deluge is not necessary.
Problems for Uniformitarians
In our experience, what appear at first as the strongest arguments against a biblical view of earth history turn out, with further scientific research, to be some of the strongest arguments against deep time. This is true also of the Williston Basin
Formation of the Williston Basin
Many theories have been put forth for how the Williston Basin could gradually subside over vast stretches of time. These tend to be highly speculative, dependent on many modeling assumptions, published eustatic curves, etc. (Fowler and Nisbet, 1984; Klein and Hsui, 1987; Quinlan, 1987; Haid, 1991; Hamdani et al., 1994; Miall, 2000; Olajide and Bend, 2014). While there is some latitude for unsteadiness in the rate of subsidence for most of the formations, belief in the swamp theory of coal formation means that the late period of subsidence in which the Fort Union sediments were deposited must have remained precisely at an elevation that maintained a close correspondence between the water table and ground surface so that lateral migration of swamps was slow enough for organic material to adequately accumulate (Moore, 1976). Thick and extensive coal seams in particular require this precise and improbable balance for long periods of time.
Preposterously slow sedimentation?
While time is often viewed as the "hero of the plot" for evolutionists, it is no friend at all. For example, Peppe et al. (2011) used paleomagnetism and chronostratigraphy to assert a duration of deposition for the Bullion Creek Formation of 1.00 to 1.25 million years. For the measured section in southwestern North Dakota, this results in an average rate of deposition of 0.15 to 0.18 mm/yr. Sonnenberg (2017) estimates 20 million years were needed to deposit the Three Forks and Bakken Formations, resulting in a maximum average rate of about 0.002 mm/yr. If we take 5,000 m of central basin sediments and divide by the half a billion years (middle Cambrian age at base), the average rate of deposition for the basin would be approximately 0.03 mm/yr. For comparison, the particle size boundary between silt and sand is 0.075 mm. So the average rate of deposition would be less than half a silt grain thick per year! Since this makes no sense in light of observable sedimentation processes, especially if sands or gravels are involved, it becomes necessary to invoke innumerable small-scale paraconformities (which must be inferred ad hoc since strata appear conformable) to account for the nearly universal “missing” time. Miall (2014) has made a valiant attempt to save uniformitarianism in this way, but as Reed (2002a; 2002b; 2004, 2016, 2018), for one, has pointed out, most of uniformitarian time is "dead time.” How such enormous amounts of “dead time” could pass without doing violence to laterally extensive deposits–if not obliterating them entirely–is baffling at best.
The phenomenon of gravel-capped planation surfaces
In the northwest part of the Williston Basin is a gravel-capped planation surface, called the Flaxville surface (Figure 13). It represents one of more than four or five planation surfaces in Montana, Alberta, and Saskatchewan, east of the continental divide (Klevberg and Oard, 1998; Oard and Klevberg, 1998). The Flaxville surface crops out as a belt of plateaus within an area 300 km east-west by 80 km north-south in northeast Montana. The plateaus were likely once continuous but later dissected. The surfaces are capped with a predominantly quartzite gravel stratum that ranges in thickness from 1 m to as much as 30 m. Percussion marks are common on the gravel. Based on paleocurrent directional indicators and the closest major source, the quartzite gravel was transported up to 700 km from central Idaho. This combination of planation and gravel transport (Oard and Klevberg, 1998; Oard, 2018) is a diluvial signature, as no present process can perform this geologic work.
Gravels north of the Little Missouri River
Evidence of glaciation is largely confined to areas north of the Little Missouri River (Fisher, 1953). However, south of the Missouri River, glacial deposits are thin and spotty and sometimes appear to simply drape over previous fluvial deposits (Neven, 1946), such as at Sperati Point in the north unit of Theodore Roosevelt National Park (Biel and Gonzalez, 2001). Predictably, these are attributed to the “ancestral Little Missouri River,” which from the mapped extent of deposits, (Murphy and Gonzalez, 2003), must have meandered over an enormous flood-plain. Klevberg has spent time investigating the largest gravel deposit in McKenzie County—the Cartwright Gravels east of the Yellowstone River, which are nearly pure quartzite with only a few crystalline rocks near the top of the deposit. The nearest potential source area for the quartzite is about 800 km west in central Idaho (Oard et al., 2005).
Gravels of southwestern North Dakota
Most surficial gravel in the study area is angular clinker that appears to have formed in place when coal seams burned. The surficial gravel deposits of interest to this study are those that show evidence of transport (Figure 14), sometimes clearly from at least as far as the western Rocky Mountains about 300 km to the west (Figure 3).
Sand and gravel unconformably cap some buttes and other scattered, isolated exposures of the Sentinel Butte Formation:
These sand and gravel deposits consist of moderately sorted, iron-stained, locally iron-cemented material of local and southwestern affinity. They contain pebbles and cobbles of well-cemented, locally-derived material (mudstone, sandstone, concretions, silicified wood, flint, silcrete, clinker, and chalcedony), and lesser quantities of quartzite and porphyry. The sand and gravel commonly have a transparent, shiny coating, probably of iron-manganese oxides (Biek and Gonzalez, 2001, p. 31).
Klevberg (unpublished information) has observed veneers of small pebbles (rocks between 4.75 mm and 75 mm—i.e. 3/16 to 3 inches—intermediate axis) north of Sentinel Butte in Golden Valley County (Figure 14A) and larger pebbles north of White Butte in Slope County (Figure 14B). Exotic lithologies predominate. He also observed a most unusual pebble veneer consisting of an estimated 80 percent agate on the summit of Sentinel Butte (Figures 14C and 14D). Agate (or transluscent chalcedony) is common in the gravels of the Yellowstone River in Montana (Figure 3) 70 km west-northwest of Sentinel Butte (Figure 4). Klevberg has observed diminutive, isolated patches of quartzite pebble veneers in McKenzie County in the badlands south of the Little Missouri River and Watford City (Figure 4).
Quartzite rocks found on plateaus in Theodore Roosevelt National Park
During Flood runoff while continents and mountains were rising and valleys and ocean basins sinking (Psalm 104:6–9), we would expect soft rocks to be pulverized to fine particles during erosion, and resistant rocks would be spread long distance from their sources. We indeed see such a signature associated with mountain ranges (Oard and Klevberg, 2005; Oard, 2008, 2011, 2013b). We have examined this pattern in particular in the northwest United States and adjacent Canada.
In the northwest states, "high-grade" metaquartzite is seen as layers mostly in central and northern Idaho, extreme western Montana, and adjacent southeast British Columbia. They are part of the Belt Supergroup, which are mostly metamorphosed shale, argillite, with minor proportions of limestone and quartzite. There are outcrops of quartzite in the Little and Big Belt Mountains of Central Montana. There is also "low-grade" quartzite, essentially a hard, silica cemented sandstone, that outcrops in the eastern Rocky Mountains. None of these latter sources is large enough to provide the many billions, if not trillions, of well-rounded quartzite rocks found east of the Continental Divide.
The quartzite presumably started as a very pure, fine quartz sandstone, quartz arenite, a sandstone formed in only small locations today but extensive in Precambrian and Paleozoic rocks (Pettijohn et al., 1987). As burial continued, the rock became hotter, possibly over 500 C (Sanders et al., 1976), and the quartz arenite metamorphosed to quartzite. In this metamorphic process, the sandstone recrystallized and its pores filled in, forming a dense, hard rock. Breaking a quartzite open with a rock hammer reveals its vitreous or glassy texture (Figure 15).
We infer that during the Recessional Stage of the Genesis Flood (Walker, 1994), the Rocky Mountains uplifted thousands of meters, exposing the quartzite to erosion. We have documented the spread of resistant, well-rounded quartzites from the outcrops of the western Rockies, as far as 700 km west to the Pacific Ocean (Oard et al., 2006), about 600 km southeast to the eastern Bighorn Basin of north-central Wyoming, and up to 1,200 km east and northeast into southwestern Saskatchewan, southwestern Manitoba, and western North Dakota (Oard et al., 2005). Nearly all of these potential sources are hundreds of kilometers away, beyond the limits of the small-scale map of Figure 13. What is significant about quartzite is that it is a very hard, tough lithology capable of enduring abrasion and impact (as indicated by percussion marks) over great distances. Few other lithologies would survive the trip. In addition, the potential source areas of the quartzite are far outside the areas where these gravels are found, indicating long-distance transport. Important erosional remnants of the Cypress Hills and Flaxville gravel sheets are shown on Figure 13.
The quartzite rocks accumulated thousands of meters deep in paleovalleys, such as east and northeast of Jackson, Wyoming, and west of Spencer, Idaho (on Interstate 15 just south of the Montana/Idaho border). They are found on the tops of the Blue Mountains of central Oregon, the tops of the Wallowa Mountains of northeast Oregon (Figure 16), on top of the deformed lava ridges of south-central Washington, on top of the Gravelly Range of southwest Montana, and on top of a flat-topped mountain in the northern Teton Range of northwest Wyoming (source of Figure 17). Such mountaintop occurrences demonstrate the spread of quartzite before the uplift of some ranges in the northwest United States.
We have also analyzed the well-rounded quartzite conglomerate cap on the Cypress Hills of southeast Alberta and southwest Saskatchewan (Oard and Klevberg, 1998). The Cypress Hills are a slightly dissected planation surface about 120 km east-west and 20 km north-south that is about 760 m above the rivers to the north and south (Figure 13). It is a high plateau, which is capped with an average of 23 m of well-rounded, iron-stained quartzite rocks that range up to small boulder size. These quartzites were transported from central Idaho (Leckie and Cheel, 1989), about 400 km away, and on the other side of the Continental Divide (which predates these gravels), based on the nearest in situ lithologically similar high-grade quartzite body. Moreover, these quartzite rocks are covered with dozens to hundreds of percussion marks, similar to other quartzite locations, indicating powerful rock-on-rock impacts during transport (Figure 18). This would require large, fast-flowing currents. Such capping quartzite and the subsequent great erosion of the high plains that followed, leaving the Cypress Hills as a high, erosional remnant, cannot be formed by any commonplace mechanism but could by diluvial runoff (Klevberg and Oard, 1998).
So, it was not unexpected that we would find quartzite in the badlands of North Dakota. And indeed, we discovered it on a terrace just east of the "petrified forest" (Figure 19). It is interesting that we did not find any on slightly lower terraces, perhaps because these formed by less energetic, more channelized flow. The quartzites were rounded, small, and mixed in with considerable fines. The largest quartzite rock seen was about 10 cm long (Figure 20), large enough to require a significant depth of flood flow on this low slope though considerably smaller than rocks observed at higher elevations near the Continental Divide. Quartzites were also found on top of nearby Sentinel Butte, which is about 240 m above the top of the terraces in the badlands, indicating at least this much erosion in southwest North Dakota. (Other evidence indicates much more than 240 m of erosion of the area.) It is also interesting that only about 20% of the rocks on top of Sentinel Butte are quartzites, while 80% were agates from an unknown source to the west. While the Cypress Hills and Flaxville Plain are capped by continuous sheets of coarse gravels of rather uniform, polished quartzite, the North Dakota remnants are small areas widely separated, typically pebble size rocks of more mixed lithologies and generally a bit more worn (mechanically weathered) on their surfaces. Some of the deposits appear to be reworked from Cypress/Flaxville sources.
Significance of the surficial gravels
The surficial quartzite and other gravel are evidence of powerful, regional currents and therefore powerful evidence for the runoff of the Flood-waters from the rising mountains and continents (Oard and Klevberg, 1998). This dispersion of resistant rocks occurred during the time “Cenozoic” strata were deposited in this area, mainly the mid-to-late Cenozoic (Oard, 2018), and the distances of transport, plus other characteristics, are strong evidence against that event being related to either uniformitarianism or postdiluvial catastrophism.
The spread of resistant rocks from uplifted mountain ranges of the western Rocky Mountains is one of dozens of indications that the Flood/post-Flood (diluvial/postdiluvial or D/P) boundary is in the Late Cenozoic, defined as the Miocene, Pliocene, and Quaternary (Oard, 2014; Clarey, 2017), though evidence of simultaneity has been observed (Oard and Klevberg, 1998). While there may have been pulses or stages in this process, there is no evidence of the erosion that would occur with a significant hiatus in the flooding, only of regional or larger flows deep enough and strong enough to transport these rocks for hundreds of kilometers and plane the landscape Why the differences in uniformitarian ages for what is clearly the same event?. The most logical explanation is that we cannot rely on their dating criteria to correlate with the timescale of Biblical Earth history (Oard, 2019). Based on numerous criteria, we might discover the D/P boundary is in the early Pleistocene at one location but in the Miocene at another location, if we assume the imprecise geological column. Each location needs to be evaluated on its own merits.
The problem of fossilization
Fossils, in particular fossil wood, pose great challenges for ancient-Earth views. We will address the problem of silicification in Part II of this series.
Acknowledgements
Oard thanks Julie von Vett for calling his attention to the ‘petrified forests’ in the badlands of southwest North Dakota. Klevberg thanks his employer for use of the company truck in roaming western North Dakota over several seasons and coworker Jim Hiersche assisted with work inside the North Unit of Theodore Roosevelt National Park, including Figure 10. Reviewers provided helpful criticisms. Deum laudamus (Psalm 46:8).
Glossary
carbonate - rock containing more than half calcite, CaCO3, dolomite, Ca,Mg(CO3)2, or other carbonate compounds. Limestone is a rock consisting of more than half calcite, and dolostone consists of more than half dolomite.
clast – an individual rock or particle.
diluvial – pertaining to or caused by the Deluge, the global flood described in Genesis 7-8.
diluvialist – one who believes the Deluge was the salient event of geologic history.
eustasy - global cycles in sea level, the fundamental assumption behind sequence stratigraphy.
gravel – unconsolidated earth material consisting of various combinations of pebbles (4.75 – 75 mm b-axis), cobbles (75 - 256 mm), and boulders (larger than 256 mm).
Laramide orogeny - period of mountain building in Western U.S., believed by evolutionists to be about 75 to 50 million years ago, and believed by many creationists to be part of the diluvial transition from “waters prevailing” to emergence of the land and the end of the Genesis Flood (cf. Psalm 104).
percussion mark – ring-shaped surficial crack in clast surface indicating violent collision. Seldom observed forming in modern environments.
planation surface – erosion surface that is quite flat and typically discordant with structure, indicating catastrophic erosion (most evident where soft and hard strata are planed alike into a flat surface).
sequence stratigraphy - approach to correlating geologic units based on eustasy and transgressive-regressive cycles (see Klevberg, 1999; 2000a; 2000b).
subcrop – rock not exposed at the surface, accessible only to subsurface sampling methods.
Walther's Law – the idea that sedimentary facies reflect the same lateral associations of environments as those observed in the present world. For example, mudstones and sandstones may be interpreted as an estuary and river channel, or delta splays and overbank deposits.
References
CRSQ: Creation Research Society Quarterly
Allen, D. 1996. Sediment ransport and the Genesis Flood–Case studies Including the Hawkesbury Sandstone, Sydney. Journal of Creation 10(3):358–372.
Anderson, F.J. 2009. Depth to Precambrian basement rock in North Dakota, North Dakota Geological Survey Geologic Investigations No. 85. North Dakota Geological Survey, Bismarck, ND.
Bailey, R.J., and D.G. Smith. 2010. Scaling in stratigraphic data series: Implications for practical stratigraphy. First Break 28(1):57–66.
Barnhart, W.R. 2011. Hurricane Katrina splay deposits: Hydrodynamic constraints on hyperconcentrated sedimentation and implications for the rock record. CRSQ 48(2):123–146.
Barnhart, W.R. 2012a. A hydrodynamic interpretation of the Tapeats Sandstone–part I: Basal Tapeats. CRSQ 48(4):288–311.
Barnhart, W.R. 2012b. A hydrodynamic interpretation of the Tapeats Sandstone–part II: Middle and Upper Tapeats. CRSQ 49(1):19–42.
Biek, R.F., and M.A. Gonzalez. 2001. The Geology of Theodore Roosevelt National Park, Billings and McKenzie Counties, North Dakota. North Dakota Geological Survey Miscellaneous Series No. 86. North Dakota Geological Survey, Bismarck, ND.
Catuneanu, O., W.E. Galloway, C.G.St.C. Kendall, A.D. Miall, H.W. Posamentier, A. Strasser, and M.E. Tucker. 2011. Sequence stratigraphy: Methodology and nomenclature. Newsletters on Stratigraphy 44(3):173–245.
Christie-Blick, N., and N.W. Driscoll. 1995. Sequence Stratigraphy. Annual Review of Earth and Planetary Sciences 23(1):451–478.
Christman, C. 1948. The Fort Union Formation of Montana and Adjacent Areas. Unpublished thesis, Montana School of Mines, Butte, MT.
Clarey, T.L. 2017. Local catastrophes or receding Floodwater? Global geologic data that refute a K-Pg (K-T) Flood/post-Flood boundary. CRSQ 54(2):100–120.
Clarey, T.L. 2020. Carved in Stone: Geological Evidence of the Worldwide Flood. Institute for Creation Research, Dallas, TX.
Clarey, T.L., and D.J. Werner. 2017. The sedimentary record demonstrates minimal flooding of the continents during Sauk deposition. Answers Research Journal 10:271–283.
Clarey, T.L., and D.J. Werner. 2020. Compelling evidence for an Upper Cenozoic Flood/post-Flood Boundary: Paleogene and Neogene marine strata that completely surround Turkey. CRSQ 56(2):68–75.
Clayton, L., C.J. Carlson, W.L. Moore, G. Groenewold, F.D. Holland, Jr., and S.R. Moran. 1977. The Slope (Paleocene) and Bullion Creek (Paleocene) Formations of North Dakota. North Dakota Geological Survey Report of Investigation No. 59. North Dakota Geological Survey, Bismarck, ND.
Dott, Jr., R.H. 2014. Rock stars: Laurence L. Sloss and the sequence stratigraphy revolution. GSA Today 23(3):24–26.
Fisher, S.P. 1953. Geology of West Central McKenzie County, North Dakota. North Dakota Geological Survey Report of Investigation 11. North Dakota Geological Survey, Bismarck, ND.
Fowler, C.M.R., and E.G. Nisbet. 1985. The subsidence of the Williston Basin. Canadian Journal of Earth Sciences 22(3):408-415.
Haid, J.H. 1991. Tectonic subsidence of the Williston Basin (M.Sc. thesis), University of Saskatchewan, Saskatoon, SK.
Hamdani, Y., J.-C. Mareschal, and J. Arkani-Hamed. 1994. Phase Change and Thermal Subsidence of the Williston Basin. Geophysical Journal International 116(3):585-597.
Hayward, A. 1985. Creation and Evolution: Rethinking the Evidence From Science and the Bible. Bethany House Publishers, Minneapolis, MN.
Jacob, A.F. 1975. Criteria for differentiating the Tongue River and Sentinel Butte Formations (Paleocene), North Dakota. North Dakota Geological Survey Report of Investigation 53. North Dakota Geological Survey, Bismarck, ND.
Klein, G. deV., and A.T. Hsui. 1987. Origin of cratonic basins. Geology 15(12):1094-1098.
Klevberg, P. 1999. The philosophy of sequence stratigraphy-part I: Philosophic background. CRSQ 36(2):72–80.
Klevberg, P. 2000a. The philosophy of sequence stratigraphy-part II: Application to stratigraphy. CRSQ 37(1):36–46.
Klevberg, P. 2000b. The philosophy of sequence stratigraphy-part III: Application to sequence stratigraphy. CRSQ 37(2):94–104.
Klevberg, P., and Oard, M.J. 1998. Paleohydrology of the Cypress Hills Formation and Flaxville Gravel. In Walsh, R.E. (editor). 1998. Proceedings of the Fourth International Conference on Creationism, technical symposium sessions, pp. 361-378. Creation Science Fellowship, Pittsburgh, PA.
Leckie, D.A., and R.J. Cheel. 1989. The Cypress Hills Formation (Upper Eocene to Miocene): A semi-arid braidplain deposit resulting from intrusive uplift. Canadian Journal of Earth Sciences 26(10):1,918–1,931.
LeFever, J.A. 1991. History of oil production from the Bakken Formation, North Dakota. In Hanson, W.B. (editor.), 1991 Guidebook to Geology and Horizontal Drilling of the Bakken Formation. Montana Geological Society, Billings, MT.
Luther, M.R., R.E. Bassler, and H. Jirges. 1995. Shaded Relief of North Dakota, one plate at 1:1,000,000 scale. North Dakota Geological Survey MM-32. North Dakota Geological Survey, Bismarck, ND.
Miall, A.D. 2000. Principles of Sedimentary Basin Analysis, Third Edition. Springer-Verlag, Berlin/Heidelberg, Germany.
Miall, A.D. 2014. Updating uniformitarianism: Stratigraphy as just a set of “frozen accidents.” In Smith, D.G., R.J. Bailey, P.M. Burgess, and A.J. Frasier (editors), Strata and Time: Probing the Gaps in our Understanding, pp. 11-36. Special Publication 404, Geological Society, London, UK.
Milana, J.P., and K-W. Tietze. 2007. Limitations of sequence stratigraphic correlation between marine and continental deposits: A 3D experimental study of unconformity-bounded units. Sedimentology 54(2):293–316.
Moore, W.L. 1976. The stratigraphy and environments of deposition of the Cretaceous Hell Creek Formation (reconnaissance) and the Paleocene Ludlow Formation (detailed), Southwestern North Dakota. North Dakota Geological Survey Report of Investigation No. 56. North Dakota Geological Survey, Bismarck, ND.
Morton, G. 2001. The geologic column and its implications for the Flood. https://chem.tufts.edu/science/Geology/GeologicColumn.htm. (accessed April 22, 2020).
Murphy, E.C., and M.A. Gonzalez. 2003. Surface geology, Sperati Point Quadrangle, North Dakota. North Dakota Geological Survey 1:24,000 Series Maps. North Dakota Geological Survey, Bismarck, ND.
Neven, C. 1946. The Keene Dome, Northeast McKenzie County, North Dakota. North Dakota Geological Survey Bulletin 21, Part I. North Dakota Geological Survey, Bismarck, ND.
Oard, M.J. 2008. Long-distance Flood transport of the Nenana Gravel of Alaska–Similar to other gravels in the United States. CRSQ 44(4):264–278.
Oard, M.J. 2011. Retreating Stage formation of gravel sheets in south-central Asia. Journal of Creation 25(3):68–73.
Oard, M.J. 2013a. Large cratonic basins likely of impact origin. Journal of Creation 27(3):118–127.
Oard, M.J. 2013b, (ebook). Earth's Surface Shaped by Genesis Flood Runoff. http://Michael.oards.net/GenesisFloodRunoff.htm.
Oard, M.J. 2014. The Flood/post-Flood boundary is in the Late Cenozoic with little post-Flood catastrophism. http://michael.oards.net/PostFloodBoundary.htm.
Oard, M.J. 2018. Flood processes into the Late Cenozoic: part 5—Geomorphological evidence. Journal of Creation 32(2):70–78.
Oard, M.J. 2019. The Deep Time Deception: Examining the Case for Millions of Years. Creation Book Publishers, Powder Springs, GA.
Oard, M.J., J. Hergenrather, and P. Klevberg. 2005. Flood transported quartzites–east of the Rocky Mountains. Journal of Creation 19(3):76–90.
Oard, M.J., J. Hergenrather, and P. Klevberg, 2006. Flood transported quartzites: part 2–west of the Rocky Mountains. Journal of Creation 20(2):71-81.
Oard, M.J., and J.K. Reed. 2017. How Noah’s Flood Shaped Our Earth. Creation Book Publishers, Powder Springs, GA.
Oard, M.J., and P. Klevberg. 1998. A Diluvial interpretation of the Cypress Hills Formation, Flaxville gravel, and related deposits. In Walsh, R.E. (editor). 1998. Proceedings of the Fourth International Conference on Creationism, technical symposium sessions, pp. 421-436. Creation Science Fellowship, Pittsburgh, PA.
Oard, M.J., and P. Klevberg, P. 2005. Deposits remaining from the Genesis Flood: Rim gravels in Arizona. CRSQ 42(1):1–17.
Olajide, O., and S.L. Bend. 2014. 1D petroleum systems modelling: An application of McKenzie’s lithospheric stretching technique. Search and Discovery #41282. https://www.searchanddiscovery.com/pdfz/documents/2014/41282olajide/ndx_olajide.pdf.html (posted March 10, 2014).
Peppe, D.J., K.R. Johnson, and D.A.D. Evans. 2011. Magnetostratigraphy of the Lebo and Tongue River Members of the Fort Union Formation (Paleocene) in the northeastern Powder River Basin, Montana. American Journal of Science 311(10):813–850.
Peterson, J.A. 1988. Geologic Summary and Hydrocarbon Plays, Williston Basin, Montana, North and South Dakota, and Sioux Arch, South Dakota and Nebraska, U.S. U.S. Geological Survey Open-File Report 87-450-N. United States Geological Survey, Washington, D.C.
Pettijohn, F.J., P.E. Potter, and R. Siever. 1987. Sand and Sandstone, Second Edition. Springer-Verlag, New York, NY.
Quinlan, G. 1987. Model of subsidence mechanisms in intracratonic basins, and their applicability to North American examples. In Beaumont, C., and A.J. Tankard, editors. 1987. Sedimentary Basin and Basin-Forming Mechanisms, pp. 463-481. Canadian Society of Petroleum Geologists Memoir 12, AGS Special Publication 5, Calgary, AB.
Reed, J.K. 2002a. Time warp I: The Permian-Triassic boundary in the Texas Panhandle. CRSQ 39(2):116–119.
Reed, J.K. 2002b. Time warp II: Basalt flows at the Midcontinent Rift System. CRSQ 39(3): 194–197.
Reed, J.K. 2004. Time warp III: The Proterozoic-Paleozoic hiatus in the northern Midcontinent. CRSQ 39(2):116–119.
Reed, J.K. 2016. Changing paradigms in stratigraphy, “A quite different way of analyzing the record.” Journal of Creation 30(1):83-88.
Reed, J.K. 2018. Changing paradigms in stratigraphy—Another new uniformitarianism? Journal of Creation 32(3):64-73.
Reed, J.K., and M.J. Oard. 2017. Not enough rocks: The sedimentary record and Earth’s past. Journal of Creation 31(2):84-93.
Sanders, J.E., A.H. Anderson, Jr., and R. Carola. 1976. Physical Geology. Harper & Row Publishers, New York, NY.
Sonnenberg, S.A. 2017. Sequence stratigraphy of the Bakken and Three Forks Formations, Williston Basin, U.S.A. Presentation given at American Association of Petroleum Geologists Rocky Mountain Section Annual Meeting, Billings, Montana, June 25-28, 2017. Posted at searchanddiscovery.com/documents/2017/10990sonnenberg/ndx_sonnenberg.pdf.
Stanton, T.W. 1910. Lance Formation. In Lerud, J. 1982. Lexicon of Stratigraphic Names of North Dakota. North Dakota Geological Survey Report of Investigation No. 71, Bismarck, ND.
Walker, T. 1994. A Biblical geological model. In Walsh, R.E. (editor). 1994. Proceedings of the Third International Conference on Creationism, technical symposium sessions, pp. 581-592. Creation Science Fellowship, Pittsburgh, PA.
Williams, E.L. 1996. The log that has been through more than one fossilization «mill.” CRSQ 33(1):5.
Wilson, R.C.L. 1998. Sequence stratigraphy: A revolution without a cause? In Blundell, D.J., and A.C. Scott (editors). 1998. Lyell: the Past is the Key to the Present, pp. 303-314. Geological Society Special Publications 143, The Geological Society of London, London, UK.
Zhu, C., and Z. Hajnal. 1993. Tectonic development of the northern Williston Basin: A seismic interpretation of an east-west regional profile. Canadian Journal of Earth Sciences 30(3):621–630.
Figures
Figure 1. The “big four shale oil basins” of the United States:
1. Williston Basin (target formations Bakken, Three Forks)
2. Denver-Julesburg (target formation Niobrara)
3. Permian (target formations/groups Wolf Camp, Spraberry, Bone Spring)
4. West Gulf (target formations Eagle Ford Group)
Figure 2. Relation of Williston Basin to nearby structures. Fault-controlled anticlines are found within the basin as well as to the west (Laramide effects). Area of darker hatching is central (deeper) part of basin. Cross section indicated by line A-A’ is shown in Figure 5.
Figure 3. The study area is the vicinity of Medora and Watford City in western North Dakota, which mark the south and north extent, respectively, of Theodore Roosevelt National Park and the North Dakota badlands.
Figure 2. The Williston Basin is one of the notable sedimentary basins of the world. Medora, North Dakota, is located near the southern edge of the Williston Basin.
Figure 4. Shaded relief map of part of western North Dakota where badlands are located. Theodore Roosevelt National Park South Unit is located immediately north of Medora, and the North Unit is located 15 miles (24 km) south of Watford City. Main drainage is Little Missouri River. Valley southwest of Watford City is drained by Red Wing Creek. Base from Luther et al. (1995).
Figure 5. Southwest-northeast cross section through Williston Basin along line indicated on Figure 2. Figure modified from Peterson (1988). Charles Formation and Spearfish Formation Fine Salt and Dunham Salt Members indicated by dashed white lines.
PRBPowder River Basin
MCA Miles City Arch
CCA Cedar Creek Anticline
NA Nesson Anticline
y Yellowstone River
m Missouri River
1 Metamorphic basement
2 Deadwood Formation
3 Winnepeg Group (Black Island, Ice Box, and Roughlock Formations)
4 Red River, Stony Mountain, and Stonewall Formations, undivided
5 Interlake Formation
6 Winnepegosis Formation
7 Prairie Formation (Prairie Salt)
8 Manitoba Group (Dawson Bay and Souris River Formations)
9 Jefferson Formation (Duperow and Birdbear Members)
10 Three Forks Formation
11 Bakken Formation
12 Madison Group (Lodgepole, Mission Canyon, and Charles Formations; Charles indicated by dashed line)
13 Big Snowy Group (Kibbey, Otter, and Heath Formations)
14 Amsden Group (Tyler, Alaska Bench, and Devil’s Pocket Formations; eastward may be referred to as Tyler, Amsden, and Minnelusa Formations)
15 Opeche and Minnekahta Formations, undivided
16 Spearfish Formation (Fine Salt and Dunham Salt Members indicated by dashed lines)
17 Ellis Group (Piper, Rierdon, and Swift Formations; strata assigned to Morrison Formation included)
18 Dakota Group (Inyan Kara, Skull Creek, Newcastle, and Mowry Formations; some refer to Inyan Kara Group with Lakota, Fusion, Fall River - Dakota; Skull Creek and above assigned to Colorado Group)
19 Colorado Group (Graneros - Mowry, Belle Fourche, Greenhorn, Carlisle, and Niobrara Formations; Skull Creek and Newcastle included by some at base)
20 Montana Group (Telegraph Creek, Eagle, Claggett, Judith River - Pierre - Bearpaw, Fox Hills, and Hell Creek Formations)
21 Fort Union Group (Ludlow, Slope, Cannonball, Bullion Creek, and Sentinel Butte Formations; in Montana, Fort Union Formation with Tullock, Ekalaka, Lebo, and Tongue River Members)
Figure 6. Stratigraphic log per Figure 5. Sloss sequences (a.k.a. megasequences) indicated per U.S. Geological Survey posted sequence boundaries. Depths (right side of figure) in feet.
Figure 7. The entire sedimentary sequence in the Medora area was initially called Lance Formation (after the type locality at Lance Creek in Wyoming). Later, differences were acknowledged and the main units were termed the Fort Union Formation, with Wasatch Formation (named for type locality in Utah) including many of the upper strata. This became the Wasatch Group and contained the Golden Valley Formation (now Camels Butte Member of same) and an unnamed formation (now Bears Den Member of Golden Valley Formation) and the Sentinel Butte Formation, though other geologists referred to the “Sentinel Butte Facies” of the Tongue River Formation, since it takes experience or careful study to differentiate the Sentinel Butte Formation from the underlying strata. North Dakota Geological Survey staff pointed out in the 1970’s that the member or formation named for the Tongue River of Wyoming and Montana was correlated with the strata underlying the Sentinel Butte Formation on the basis of inferred age, with no physical connection. They therefore renamed this the Bullion Creek Formation with the type locality approximately 17 miles south-southwest of Medora. They further distinguished the underlying Slope Formation on lithologic grounds. The U.S. Geological Survey holds to the older nomenclature.
The Hell Creek Formation is famous for its dinosaur fossils, but it also contains fossils of fish, amphibians, mammals, and a diversity of plants. Many of these same fossils appear in Fort Union strata, but by definition the highest dinosaur-fossil-bearing formation is classified as Hell Creek. The Golden Valley Formation is rich in plant fossils, particularly the floating fern Salvinia and a wide spectrum of temperate and tropical plants. Fewer animal fossils are found.
Figure 8. Each day, hundreds of train passengers on Amtrak’s Empire Builder pass between the restored Fort Union and the nearby outcrop of Sentinel Butte strata (Fort Union Group), most of them staring at their screens and completely oblivious to the fine geology right outside the window. Fort Union was built two centuries ago as a trading post near the confluence of the Yellowstone River with the Missouri River. South and west of Fort Union, the relationship between the Tongue River and Bullion Creek Formations has yet to be resolved. Base oblique aerial from Google Earth, view toward the west-northwest.
Figure 9. The contact between the Bullion Creek Formation and Sentinel Butte Formation is evident just south of the “Petrified Forest” in the South Unit of Theodore Roosevelt National Park. The “Petrified Forest” abuts the Big Plateau where quartzite pebbles were observed. It is stratigraphically and topographically lower than the Big Plateau.
Figure 10. Outcrop of basal sandstone of Sentinel Butte Formation in North Unit of Theodore Roosevelt National Park. Note man by sign for scale.
Figure 11. This piece of fossilized wood was found in conjunction with a clinker bed (burned lignite) within the Sentinel Butte Formation across the Little Missouri River from the North Unit of Theodore Roosevelt National Park. Knife blade is approximately 5 cm long.
Figure 12. Snippet of geologic map of study area. Bullion Creek Formation is light gray, Sentinel Butte Formation dark gray.
Figure 13. Map of locations of surficial quartzite gravels east of the Continental Divide.
Figure 14. Gravel veneers are present in scattered locations in western North Dakota. A) Small quartzite pebbles north of Beach, North Dakota (42 km west of Medora) in Golden Valley County. Pencil shows scale. B) Quartzite pebbles up to 60 mm diameter capping small bench north of White Butte in Slope County. This location is ca. 51 km east-southeast of Medora. White Butte is the highest point in North Dakota. C) View north from summit of Sentinel Butte in Golden Valley County. D) The unusual gravel on the summit of Sentinel Butte is roughly 20 percent Sentinel Butte Formation sandstone and 80 percent agate.
Figure 15. A quartzite cobble from the Cypress Hills (“cypflax”) with abundant percussion marks. This has been broken with many blows of a sledgehammer to show a fresh surface. Quartzites with virtually no porosity form this texture that gives them the moniker “sugar agate.”
Figure 16. NATHAN OARD. Large quartzite boulder (estimated mass 200 kg) on top of a ridge at 2,500 m elevation near Lookout Mountain, Wallowa Mountains, Northeast Oregon (Nathan Oard provides scale). Photograph courtesy of Paul Kollas.
Figure 17. Well-rounded quartzite cobble from the top of Red Mountain, northern Teton Mountains, Wyoming. Note the small round pressure solution marks and fractures on the cobble, caused by rock on rock contact under high pressure of deposited quartzites in a paleovalley. Angular rock at upper left is chert.
Figure 18. Percussion marks on a weathered “cypflax” cobble. Note the density of the marks, with many overlapping other percussion marks.
Figure 19. Klevberg pointing to the Big Plateau in the distance upon which the well-rounded quartzites were found in the badlands of Theodore Roosevelt National Park (view east). Note the small, light-colored petrified trees along the slope.
Figure 20. Ten-cm long quartzite found on a terrace in South Unit of Theodore Roosevelt National Park.